Introduction
In March 2025, a devastating earthquake struck near Mandalay in Myanmar with a moment magnitude (Mw) of 7.7 at a shallow depth of 10 km UTC, severely impacting affected areas . The catastrophic event led to extensive damage—thousands of buildings collapsed building into heaps of rubble and debris—and caused high casualties, reminding us that it is not the seismic event itself, but how we build that ultimately determines its impact on people.
Earthquakes are a global threat affecting millions around the world; as the saying goes, “earthquakes don’t kill people, buildings do.” This statement underscores the importance of resilient design, especially since it is not widely published in many resources. , strict codes, and modern engineering solutions in relation to humanitarian affairs . Today’s article examines the science behind these natural events, uses Myanmar as a critical case study, and reviews advanced codes and techniques to build structures that can withstand strong shaking.
We will begin by explaining the fundamental science of earthquake processes, then analyze Myanmar’s seismic vulnerability—including details of the march 2025 quake—followed by a discussion of engineering and architectural solutions that have evolved over the year . The article concludes with practical strategies for preparedness, response, and rebuilding in the aftermath of an earthquake.
This comprehensive report is intended to educate a broad audience—including engineers, urban planners, government organizations, and the general public—about the necessity to build safer structures. Our roadmap covers the underlying science, a detailed case study of Myanmar, a review of resilient engineering methods, and finally a look into community preparedness and long-term recovery strategies.
The Science Behind the Shake
What Is an Earthquake?
An earthquake is a sudden shaking of the land caused by the release of energy stored in the Earth’s crust. This energy accumulates over time due to the slow motion of tectonic plates and is released when the stress overcomes the strength of rocks. The study of these phenomena helps engineers build safer structures to reduce wreckage in future earthquake.
Plate Tectonics Basics
The Earth’s outer shell is divided into enormous sections called tectonic plates. These plates move slowly—through convergence, divergence, or transform motion—and their interactions can cause earthquakes. Myanmar, as a country, lies at a critical junction where several plates interact, which makes it highly prone to seismic hazards. In Myanmar, the movement along the Sagaing Fault and the nearby subduction zone has historically caused significant shaking.
Faults and Energy Release
A fault is a fracture in the Earth’s land along which earthquake damage originates. The build-up of stress is released at the point called the focus (or hypocenter), directly below the surface point known as the epicenter. The energy radiates in all directions as seismic waves. In the March 2025 earthquake, the rupture occurred along a strike-slip fault in Mandalay, releasing a massive amount of energy.
Measuring the Tremors
Magnitude:
The energy released by an earthquake is measured on a logarithmic scale. The Moment Magnitude (Mw) scale is most widely used—each whole number increase represents roughly 32 times more energy. For example, the Mandalay event at 7.7 Mw released exponentially more energy than smaller events.
Intensity:
Seismic intensity, measured by the Modified Mercalli Intensity (MMI) scale, reflects the observable effects of the shaking. The March 2025 event reached an MMI of IX (Extreme) near its epicenter.
Seismic Waves:
Seismic energy travels in various forms:
- P-waves (primary waves) are the fastest and first to be detected.
- S-waves (secondary waves) move slower and cause more damage.
- Surface waves, including Love and Rayleigh waves, induce most structural damage on the surface.
What Influences Ground Shaking?
Several factors modify the ground shaking experienced during an earthquake:
- Magnitude and Depth: Larger earthquake magnitudes cause more energy release, and a shallow focus, such as the 10 km depth in March 2025, results in higher shaking intensity.
- Distance from Epicenter: As one moves away from the epicenter, the shaking effects decrease.
- Local Geology (Site Effects): The type of land and soil—especially soft soils like clay—can amplify shaking. In Myanmar, areas with reclaimed or loose soils experienced higher amplitudes, which contributed to incidents like the collapsed building in Bangkok.
- Fault Rupture Characteristics: Factors such as rupture direction, speed (including supershear motion), and fault length work together to determine the pattern of shaking.
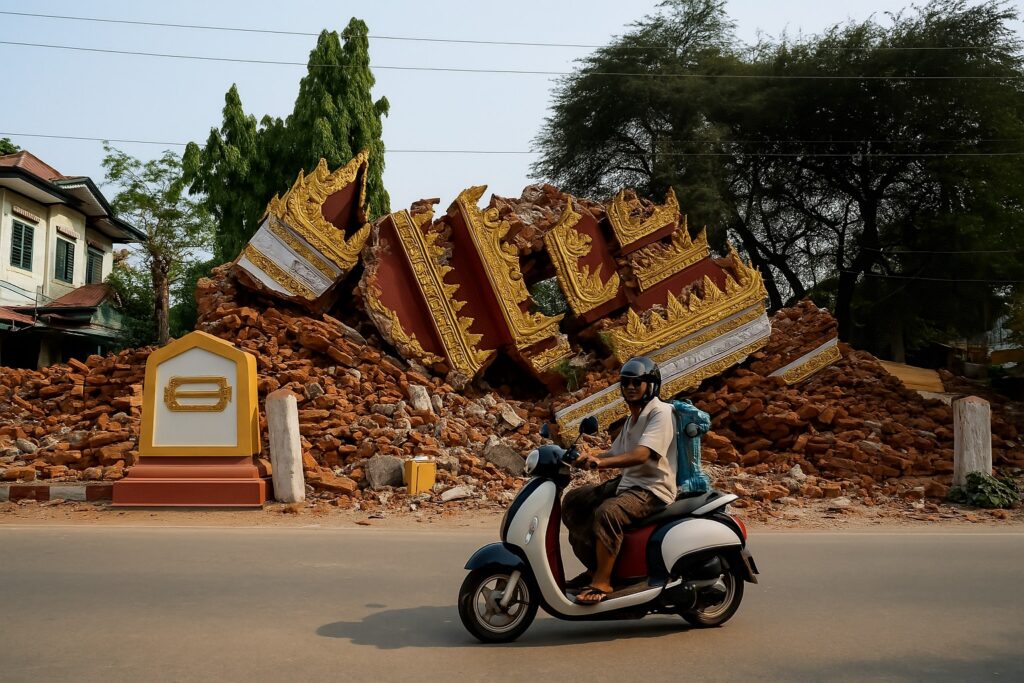
Myanmar Case Study in Seismic Vulnerability
History
Located on major fault lines—the Sagaing Fault and the Sunda megathrust—Myanmar has long been exposed to earthquake hazards, affecting many areas . Historical earthquakes prior to 2015 have left a legacy of both cultural and infrastructural damage. These past events serve as both warnings and lessons, often documented in various report . Notably, the 1931 and 1946 events remind us that seismic hazards in Myanmar are not recent phenomena.
Significant Past Events (2015-2025)
Over the past decade, Myanmar experienced several key earthquakes:
- In 2016, a 6.8 Mw event in the Magway region caused minor temple damage in Bagan.
- A 5.1 Mw shock in 2017 in Yangon resulted in damage and injuries.
- The March 2025 earthquake near Mandalay was the most catastrophic, leading to thousands of fatalities and widespread destruction.
Below is a concise table summarizing critical events during this year range:
Date | Location | Magnitude | Depth (km) | Fatalities | Injuries |
---|---|---|---|---|---|
2025-03-28 | Sagaing near Mandalay | 7.7 | 10 | 3,564+ | 4,850+ |
2023-06-07 | Ayeyarwady | 4.8 | 10 | 3 | — |
2023-05-31 | Mohnyin | 5.9 | 10 | — | — |
2022-07-21 | Kengtung | 5.9 | 5 | — | — |
2017-03-13 | Yangon | 5.1 | 10 | 2 | 36 |
2016-08-24 | Magway | 6.8 | 84.1 | 4 | 20 |
The 2025 Mandalay Earthquake (Mw 7.7)
The March 2025 earthquake in Mandalay was particularly destructive. Occurring at 06:20:54 utc, its strike-slip mechanism along the Sagaing Fault led to the failure of numerous structures. Immediate damage was widespread: many buildings collapsed, major roads were blocked by debris, and critical infrastructure like the Ava Bridge collapsed, leaving dozens of people in jeopardy.
- Immediate Impact: In Mandalay and surrounding areas, the earthquake caused catastrophic damage. Emergency rescue teams and search and rescue operations were quickly mobilized, but the scale of destruction overwhelmed local capabilities.
- Regional Consequences: Neighboring areas experienced strong shaking. In Bangkok, for example, a collapsed building resulted from the amplified vibrations due to soft, compressible soils. The earthquake was felt in India, China, and Vietnam, highlighting the regional nature of such events.
Underlying Vulnerabilities in Myanmar
The earthquake exposed numerous vulnerabilities in Myanmar’s built environment:
- Building Stock: A mix of unreinforced masonry, non-ductile concrete frames, and informal construction methods has left many structures highly susceptible to shaking.
- Codes and Construction Practices: Although codes like the Myanmar National Building Code (MNBC) (updated in 2016 and 2020) were published, enforcement is weak. The majority of buildings pre-date these codes, and poor construction quality remains rampant.
- Soil and Site Risks: Many sites in Myanmar are located in areas prone to liquefaction or landslides. In regions with soft soils, such as those that build in Myanmar and Bangkok, shaking is significantly amplified.
- Preparedness Gaps: Limited early warning systems and a lack of continuous public drills mean that people are often caught unprepared when a severe event occurs.
Engineering and Architectural Strategies for Building Resilience
A major lesson from the march 2025 earthquake is that the way we build can dramatically reduce loss of life and damage. Resilient design is not just about preventing collapse; it is about ensuring that structures remain functional to support people during and after an event, fighting against potential loss .
Core Principles of Earthquake-Resistant Design
The primary goal of seismic design is life safety, while modern approaches strive to maintain operability post-event. Key principles include:
- Ductility: The ability of a structure to deform under stress without fracturing.
- Strength and Stiffness: Ensuring sufficient load-carrying capacity while allowing controlled motion.
- Redundancy: Creating multiple load paths to prevent total collapse even if one component fails.
- Continuous Load Paths: Seamless integration between floors and vertical structural elements for even distribution of forces.
Structural Engineering Solutions
Engineers have developed methods to build a stable structural skeleton that can resist the lateral forces of an earthquake:
- Shear Walls and Braced Frames: These elements help build rigidity and resist lateral forces. Steel cross-bracing is often incorporated into these systems.
- Moment-Resisting Frames: Special design of connections (between beams and columns) ensures ductility and energy absorption during seismic events.
- Diaphragms: Floors and roofs designed to distribute lateral loads to the vertical resistance system.
Materials also play a key role:
- Reinforced Concrete: When properly designed with sufficient rebar and proper detailing (such as confining ties in columns), reinforced concrete can sustain significant damage without failing. In contrast, brittle, unreinforced concrete or masonry is prone to cracking and collapse.
- Structural Steel: Naturally ductile, steel is used in various frame systems and performs well during an earthquake.
- Timber and Mass Timber Systems: These are lightweight and offer flexibility; when designed correctly, they can perform robustly under seismic loads.
- Advanced Materials: Innovations like high-performance concrete and fiber-reinforced polymers (FRP) are used in retrofitting projects to enhance seismic performance.
Advanced Seismic Protection Systems
Modern engineering introduces additional protective systems:
Base Isolation:
This technique builds a separation between a structure and its foundation, using flexible bearings (such as lead-rubber bearings) to absorb and dissipate seismic energy. Examples include the Sendai Airport terminal in Japan and SF City Hall in the united states.
Energy Dissipation Devices (Dampers):
Viscous fluid dampers, friction dampers, and tuned mass dampers (as seen in Taipei 101) absorb seismic energy, reducing the forces transmitted to the structure.
Rocking Systems and Low-Damage Design:
Some systems allow the building to rock safely, using engineered fuses that can be replaced after an earthquake. This approach minimizes the need for extensive repairs and helps people return to normal work quickly.
Architectural Considerations for Safety
Architectural design is critical in reducing damage:
- Building Form and Configuration: Simple, regular, and symmetrical designs help evenly distribute seismic loads and reduce torsional effects. Complex shapes or irregular layouts often lead to stress concentrations, increasing vulnerability.
- Non-Structural Elements: A significant portion of injuries and damage results from falling non-structural components (ceilings, partitions, facades). Ensuring that these are securely attached can greatly reduce risks.
- Material Choices: Using lightweight roofing and cladding materials reduces the overall inertial forces during an earthquake.
- Urban Planning and Site Selection: Proper zoning and site selection—avoiding areas with high liquefaction or landslide risk—contribute greatly to the overall resilience of a community.
Retrofitting Existing Buildings and the Role of Codes
Most buildings in many Myanmar urban centers were constructed before modern codes were published. Retrofitting is essential:
- Techniques: Common approaches include adding shear walls, steel jacketing of columns, and installing energy-dissipating devices.
- Prioritization: Critical facilities such as hospitals, schools, and government buildings should be first on the list for retrofitting due to their importance during emergencies.
- Codes and Enforcement: Although codes such as the MNBC (updated in 2016 and 2020) exist, there are significant gaps in enforcement. Without rigorous inspections and maintenance, even well-designed buildings may fail during a strong earthquake.
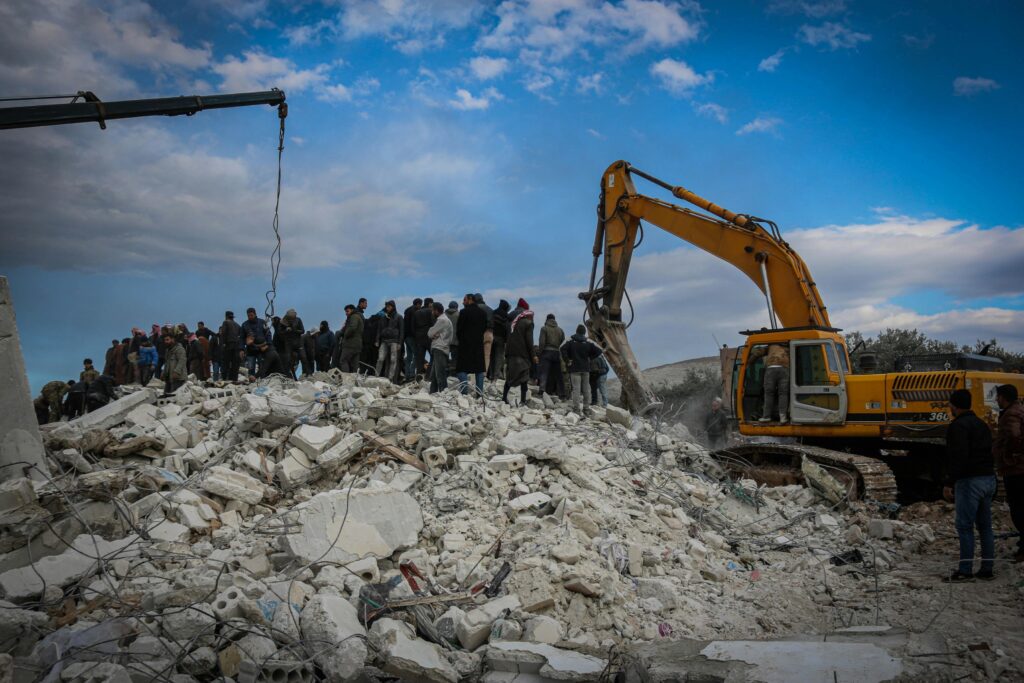
Preparedness, Response, and Recovery
Resilience extends beyond design. Preparing the public, coordinated response, and efficient recovery processes are as vital as engineering, and in addition, requires community engagement .
Community and Individual Preparedness
Awareness and education can build a resilient society, empowering groups to better prepare for disasters :
- Risk Understanding: People should know the seismic hazards in their locality and the likely damage that an earthquake could cause.
- Emergency Plans: Every household and community should have a clear plan that includes communication protocols and designated safe spots.
- Emergency Kits: Essential items such as water, food, first-aid supplies, and flashlights must be readily available.
- Securing Interiors: People can build safer homes by fastening furniture, securing water heaters, and storing heavy items securely.
- Regular Drills: Practicing “Drop, Cover, Hold On” is essential to ensure that when an earthquake occurs, responses are instinctive.
During and After the Shake
During an earthquake, the people’s defense force plays a crucial role in ensuring safety and response.
- Indoors: The best practice is to “Drop, Cover, Hold On”. Avoid windows and heavy objects.
- Outdoors: Move to open spaces away from buildings, power lines, and overpasses.
- In Vehicles: Pull over safely and avoid bridges.
After the shaking:
- Immediate Actions: People should check for injuries, inspect for gas leaks, and be cautious of falling debris or unsecured objects. Aftershocks may occur within two weeks, requiring further precaution.
- Search and Rescue Operations: Rescue teams comprising local authorities, the military, and international organizations must coordinate quickly to remove debris and extract survivors. In some areas, the armed wing of local groups and volunteer rescue efforts have been critical.
- Emergency Services: Rapid assessments of damage are essential for directing aid. Hospitals and emergency rescue teams search for survivors amidst the rubble.
- Public Coordination: The united nations and other aid organizations often step in to support local efforts when the local capacity is exceeded.
Long-Term Recovery and Rebuilding
The recovery phase after an earthquake can last for years:
- Economic Impact: Direct costs from damage and indirect costs from lost work and collaboration interruptions are extensive.
- Rebuilding Process: Decisions on how to build back must balance speed with the necessity to improve resilience. Strict adherence to updated codes is critical to prevent future collapse.
- Improved Land Use: Urban planners and organizations involved in recovery must reassess land use and avoid constructing in high-risk areas.
- Case Studies: Experiences in Japan and new Zealand offer updates to design practices after major earthquake events, showing that systematic rescue efforts and careful recovery planning can dramatically reduce future risk.
Concluding Thought
While earthquakes are a natural hazard that no society can completely avoid, their tragic impact on people can be mitigated if we build smarter, enforce rigorous codes, and prepare through coordinated emergency search and rescue operations.
The lessons from the march 2025 quake in Myanmar and other examples show that modern engineering and architectural strategies—combined with effective public policy and community preparedness—are the key to reducing damage, clearing debris, and ensuring that future events do not result in preventable loss of life.
Through continuous updates, international collaboration, and steadfast commitment, along with considerations for wind forces we can make resilient infrastructure a reality. It is a path that started with a clear understanding of the science and must continue with rigorous work, sound decisions, and unwavering focus on safety.